Science / Anaerobic Capacity: How to Increase, Exercises, Benefits
Anaerobic Capacity: How to Increase, Exercises, Benefits
Delay Lactic Acid Buildup and Muscle Fatigue
High altitude simulation is a “secret weapon” for enhanced performance. It boosts anaerobic capacity, allows you to delay muscle fatigue and lactic acid, and gives you a competitive edge that’s entirely safe and legal.
In this article we’ll explore:
- Hypoxia and hypercapnia
- What anaerobic exercise is
- What lactic acid is
- How lactic acid buildup affects athletic performance
- And how anaerobic capacity exercises produce beneficial changes in your body
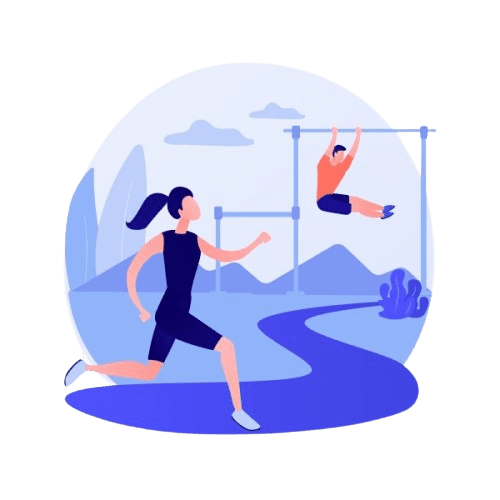
When it comes to breathing techniques, athletes tend to focus on oxygenation. Whether you’re new to sport or a seasoned pro, breathing efficiency is vital. It’s a major differentiator for competition and performance.
But, it’s during anaerobic exercise that adaptations take place. This is when you push yourself. It’s when exercise is so intense that the body turns to other means to get energy. Oxygen is out of the picture.
It’s relatively easy to increase aerobic capacity. But anaerobic capacity is different. For a start, you’d have to exercise for longer at a very high intensity. And that’s not possible for everyone. Repeated high intensity bursts can damage the body. Lactic acid burns in your muscles, and muscle fatigue strikes. You have to slow down or stop, whether you like it or not. And the harder you push, the higher your injury risk.
Fatigue is an issue for every athlete. But it’s particularly relevant in anaerobic exercise. It’s the point at which it’s physically impossible to continue at the same intensity. In chemical terms, it has to do with an increase in hydrogen ions in the blood.
Let’s begin with a quick look at breathing chemistry. This will help us understand important factors like lactic acidosis. And it will make it easier to “get” what happens during your anaerobic workout.
Breathing Chemistry and Blood pH in Sports Physiology
Breathing involves the exchange of oxygen and carbon dioxide. It is vital for life. But it’s also important for homeostasis, the maintenance of a healthy internal balance.
Breathing impacts the pH of the blood (how acid or alkaline your blood is). Normal blood pH is between 7.35 and 7.45. Even slight fluctuations can cause very serious effects. Breathing is important because the level of carbon dioxide in the blood has a direct impact on blood pH. Too much CO2, and you get respiratory acidosis (the blood becomes too acidic). Too little, you get respiratory alkalosis (the blood becomes too alkaline)
What Do All These Words Mean?
An acid is a molecule that breaks down to form hydrogen ions (H+). For example, carbon dioxide in the blood forms carbonic acid (H2CO3). Carbonic acid breaks down to form hydrogen and bicarbonate ions.
When compounds like acids break into smaller parts, scientists call it “dissociation”. Dissociation is usually reversible. Molecules split into ions, atoms and radicals. When carbonic acid dissociates, it produces hydrogen and bicarbonate. The bicarbonate acts as a “buffer.” A buffer is something that minimizes the change in pH. It works by “mopping up” hydrogen ions, so the blood doesn’t become too acidic.
We know from school chemistry lessons that pH is a measure of acidity/alkalinity. But it actually refers to the concentration of hydrogen ions. So, the concentration of H+ determines acidity/alkalinity.
How Does Hypoxic Training Work?
Simulation of high altitude training involves the practice of strong breath holds.
This causes:
- Hypoxia: blood oxygen levels drop to between 80 and 90%. Normal blood oxygen concentration is between 95 and 100%. Anything less than 90% is hypoxic.
- Hypercapnia: blood carbon dioxide (CO2) increases. When blood CO2 is higher than normal, it’s called hypercapnia.
Hypoxia and hypercapnia result in an increase in hydrogen ions. As H+ builds up, the blood acid-base balance changes. When you expose your body to a surge in H+ during training, the body adapts, reducing the onset of muscle fatigue.
When you hold the breath, CO2 can’t leave the body. It builds up in the lungs, blood and muscles (hypercapnia). It forms carbonic acid, which dissociates to H+ and bicarbonate.
When oxygen levels are normal, H+ oxidizes in the cells to form water (H20). When oxygen is low (hypoxia) oxidization can’t take place. In these “anaerobic” conditions, the H+ combines with something called pyruvic acid. Pyruvic acid is a byproduct of glycolysis – the metabolism of glucose that supplies energy to the cells. When pyruvic acid combines with hydrogen, it forms lactic acid. Lactic acid then dissociates into H+ and lactate ions. Here, lactate is the buffer.
During breath holding to simulate altitude training, the muscles become acidic. This is the main cause of adaptations that happen after the training. Scientists suggest the body develops an enhanced “buffering capacity.” Which means H+ accumulates more slowly, meaning you can keep going for longer.
What Happens During Anaerobic Exercise?
Anaerobic means “without oxygen.” So anaerobic exercise is any type of exercise that breaks down glucose for energy. Without using oxygen. Think high-intensity, short burst activities. HIIT, weightlifting, MMA, sprinting and boxing. Pushing beyond your VO2 max. The difference between anaerobic vs aerobic exercise is oxygen.
During aerobic exercise, the body uses oxygen to get energy from fat and glucose. When you train hard, your muscles experience a temporary shortage of oxygen. This means your body must get energy from a process called glycolysis, glycolytic metabolism or anaerobic metabolism. Glycolysis uses glucose stored in the muscles for energy. And it produces energy, fast.
But as we saw, it also produces lactic acid. Feel the burn.
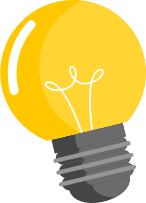
Benefits of anaerobic exercise include:
- Greater power
- Better bone mineral density
- Enhanced metabolism
- Weight management
- Less depression
- Lower risk of disease
- More energy
- Increased lactate threshold
When you practice these anaerobic capacity exercises, your body will adapt. High altitude simulation increases your body’s lactate threshold. Which means it improves your body’s tolerance to lactic acid. Muscle fatigue and lactic acid accumulation are closely related. So, a better lactate threshold means better anaerobic capacity. This delays the onset of fatigue and improves performance. It means you can train harder for longer, without risk of overtraining. It also means you can train at a more moderate pace. And still get the benefits.
What is Lactic Acid?
Lactic acid is a metabolic byproduct. We’re most familiar with it during exercise. But if there isn’t enough oxygen present, all cells produce lactic acid. This can cause something called metabolic acidosis. Lactic acidosis can produce symptoms including nausea, weakness and vomiting. Particularly in people who have a compromised liver.
Lactic acid is what causes the burning sensation in your muscles when you’re pushing hard. But to some extent, it’s misunderstood. Lactic acid isn’t always the bad guy. The body is smarter and more complex than that.
Does Lactic Acid Cause Muscle Fatigue?
When blood lactate levels are high, the muscle cells become more acidic. This makes it difficult for the body to break down glucose to get energy. The acidic environment also blocks nerve signals from the brain to the muscles. Your legs begin to feel heavy, and so you slow down.
This process might seem counterproductive. Why does the working muscle produce a substance that slows it down? The answer is that it prevents overtraining and long-term damage during extreme exercise. It protects you from permanent injury.
Once the body has slowed down, oxygen becomes available again. Lactate reverts back to pyruvate, and aerobic metabolism recommences. This gives your body the energy it needs for recovery. George Brookes, professor of integrative biology at UC Berkeley, says, “Lactate production is a strain response, it’s there to compensate for metabolic stress.“
Without this added fuel, the body would not have the energy to repair itself.
Lactic acid buildup doesn’t cause muscle soreness in the days after intensive exercise. This is contrary to what many people believe. The burning sensation causes us to stop overworking the body. It forces a period of recovery. The delayed-onset muscle soreness (DOMS) that happens two or three days later? That’s due to tiny tears in the muscle tissue. As Bulletproof’s Dave Asprey says: “Exercise is a classic example of hormesis – you damage your muscle fibers and they build back bigger and stronger.” In other words, when you push your body, it becomes more resilient. Research has shown that hypoxia is beneficial in muscle repair. Both in the formation of muscle tissue and the stimulation of muscle stem cells.
How Does Lactic Acid Affect Breathing?
Scientists have proven that exercise-induced lactic acidosis plays a role in hyperventilation. This hyperventilation starts at the respiratory compensation point (RCP). The RCP is the point at which the pressure of arterial oxygen begins to drop during intense exercise. Remember, when oxygen is absent, every cell will produce lactic acid. But this is not the only thing that stimulates breathing during heavy exercise. Sensory inputs from exercising muscles also contribute.
Lactic acid is also not the only cause of heavy legs. When the breathing muscles tire, the body diverts blood circulation from the legs. This supports breathing by redistributing oxygenated blood to where it’s most needed. Strong breathing muscles and breathing efficiency are also important in delaying muscle fatigue. You can develop your breathing muscles with high altitude simulation training.
How Do I Reduce Lactic Acid?
Scientists have proven that athletes who practice breathing exercises can increase their performance. Without increasing lactic acid buildup.
It’s also important to:
- Stay hydrated
- Breathe light for better oxygenation
- Use breathing exercises during warmup and recovery
- Restore full time nasal breathing
- Practice exercises to simulate high altitude training
Injured athletes can use the exercises to simulate high altitude to maintain condition.
How Do You Increase Your Anaerobic Threshold?
Your anaerobic threshold is your lactic acid threshold. They are two ways of describing the same thing. And better anaerobic capacity can mean the difference between winning and losing. It’s worth the effort.
Scientists have recently shown that altitude training increases lactic acid tolerance. It allows your body to handle a higher lactic acid level before getting tired. Altitude training also boosts cardiorespiratory fitness. And it improves running speed, even when lactic acid is present in the muscles.
Many people rely on elevation masks to recreate conditions at high altitude. But masks don’t work that way. They increase resistance to airflow and buildup of carbon dioxide. But they don’t reduce the pressure of oxygen.
So how can you recreate the hypoxia and hypercapnia typical of high altitudes? By integrating the Oxygen Advantage® anaerobic capacity exercises into your workout.
Anaerobic training can be hard. But the rewards are significant. With the right breathing exercises, you can experience the benefits. Without the burn.
What the Scientists Say about Anaerobic Capacity
- IMPROVED SWIMMING PERFORMANCE. IMPROVED ANAEROBIC CAPACITY.
Time performance was significantly improved in trials involving breath holding following an exhalation [100m: – 3.7 ± 3.7s (- 4.4 ± 4.0%); 200m: – 6.9 ± 5.0s (- 3.6 ± 2.3%); 400m: – 13.6 ± 6.1s (-3.5 ± 1.5%)] but did not change in CONTROLS. In breath holding following an exhalation, maximal lactate concentration (+ 2.35 ± 1.3 mmol.L-1 on average) and the rate of lactate accumulation in blood (+ 41.7 ± 39.4%) were higher at Post- than at Pre- in the three trials whereas they remained unchanged in CONTROLS.
Under these conditions, there was also an increased lactate concentration, revealing a greater glycolytic activity as compared to the same exercise performed with normal breathing. Such results, already reported in terrestrial activities, were original in swimming. Conversely, in studies in which swimmers applied hypoventilation at high lung volume (i.e. inhale-hold), that is the classical technique used since the 1970’s, no hypoxic effect occurred and lactate concentration was not different or even lower than exercise with normal breathing.
Increased Lactate max reflects an improved anaerobic capacity and may be due to a greater ability to tolerate high concentrations of lactate and high level of acidosis, as reported after high-intensity training.
Woorons X, Mucci P, Richalet JP, Pichon A. Hypoventilation Training at Supramaximal Intensity Improves Swimming Performance. Med Sci Sports Exerc. 2016 Jun;48(6):1119-28
- DELAYED APPEARANCE OF BLOOD LACTATE
Five male subjects performed 16 by 4 min cycling bouts alternating with 16 min rest periods. Breathing frequency was voluntarily controlled starting 10 s before each 3rd min of exercise and maintained throughout the rest of the exercise period. Four different breathing patterns at each exercise intensity were used: normal breathing (NB), breathing every 4 s, breathing every 8 s, and maximal Reduced Frequency Breathing.
Except for the NB trials, subjects held their breath at functional residual capacity during each breathing interval. The researchers concluded: results might indicate that reduced breathing frequency inhibited lactate removal from working muscles during exercise.
Yamamoto Y, Takei Y, Mutoh Y, Miyashita M. Delayed appearance of blood lactate with reduced frequency breathing during exercise. Eur J Appl Physiol Occup physiol. 1988;57(4):462-6.
- EXPOSING THE BODY TO INCREASED ACIDOSIS TO IMPROVE BUFFERING CAPACITY
Repeated prolonged expirations carried out down to residual volume during a submaximal exercise led to a drop of SaO2 down to 87% and was also accompanied by a marked hypercapnia. The severe arterial desaturation was caused by a PAO2 decrement, a widened PAO2 − PAO2 and a right shift of ODC.
We also reported a greater VO2 and fh during PE than NB70 may be caused by the greater activity of the respiratory muscles and the adrenergic system. Finally, the prolonged expiration led to a greater blood acidosis, mainly hypercapnic and possibly also linked to a greater muscle acidosis.
Xavier Woorons, Pascal Mollard, Aur´elien Pichon, Alain Duvallet, Jean-Paul Richalet, Christine Lamberto. Prolonged expiration down to residual volume leads to severe arterial hypoxemia in athletes during submaximal exercise. Respiratory Physiology & Neurobiology 158 (2007) 75–82
- BLOOD ACIDOSIS WAS REDUCED AND THE OXIDATIVE STRESS NO LONGER OCCURRED
Repeated epochs of breath-holding were superimposed to the regular training cycling program of triathletes to reproduce the adaptative responses to hypoxia, already described in elite breath-hold divers [Respir. Physiol. Neurobiol. 133 (2002) 121].
After training, the duration of static apnea significantly lengthened and the associated bradycardia was accentuated; we also noted a reduction of the post-apnea decrease in venous blood pH and increase in lactic acid concentration, and the suppression of the post-apnea oxidative stress (increased concentration of thiobarbituric acid reactive substances).
After dynamic apnea, the blood acidosis was reduced and the oxidative stress no more occurred. These results suggest that the practice of breath-holding improves the tolerance to hypoxemia independently from any genetic factor.
Joulia F, Steinberg JG, Faucher M, Jamin T, Ulmer C, Kipson N, Jammes Y.Breath-hold training of humans reduces oxidative stress and blood acidosis after static and dynamic apnea. Respir Physiol Neurobiol. 2003 Aug 14;137(1):19-27.
- REDUCED OXIDATIVE STRESS AND BLOOD LACTIC ACIDOSIS IN TRAINED BREATH-HOLD HUMAN DIVERS
We hypothesized that the repetition of brief epochs of hypoxemia in elite human breath-hold divers could induce an adaptation of their metabolic responses, resulting in reduced blood acidosis and oxidative stress.
Trained divers who had a 7-10 year experience in breath-hold diving, and were able to sustain apnea up to 440 sec at rest, were compared to control individuals who sustained apnea for 145 sec at the most. The subjects sustained apnea at rest (static apnea), and then, performed two 1-min dynamic forearm exercises whether they breathed (control exercise) or sustained apnea (dynamic apnea).
In divers, the changes in lactic acid, TBARS, RAA, and GSH concentrations were markedly reduced after static and dynamic apnea, as well as after control exercise. Thus, human subjects involved in a long duration training programme of breath-hold diving have reduced post-apnea as well as post-exercise blood acidosis and oxidative stress, mimicking the responses of diving animals.
Joulia F, Steinberg JG, Wolff F, Gavarry O, Jammes Y. Reduced oxidative stress and blood lactic acidosis in trained breath-hold human divers. Respir Physiol Neurobiol. 2002 Oct ;133(1-2):121-30.
- IMPROVED SPRINT ABILITY IN SWIMMING THROUGH ENHANCED ANAEROBIC GLYCOLYSIS
Repeated-sprint training in hypoxia has been shown as an efficient method for improving repeated sprint ability in team-sport players but has not been investigated in swimming.
Repeated sprint training in hypoxia induced by voluntary hypoventilation at low lung volume improved repeated sprint ability in swimming, probably through enhanced anaerobic glycolysis. This innovative method allows inducing benefits normally associated with hypoxia during swim training in normoxia.
Trincat L, Woorons X, Millet GP. Repeated Sprint Training in Hypoxia Induced by Voluntary Hypoventilation in Swimming. Int J Sports Physiol Perform. 2016 Jun 13.
- LOWER MUSCLE OXYGENATION AND HIGHER BLOOD LACTATE CONCENTRATION: ROLE OF HYPOXIA AND HYPERCAPNIA
This study demonstrated that exercise with voluntary hypoventilation induced a lower tissue oxygenation and a higher [La] than exercise with normal breathing. This was caused by a severe arterial O2 desaturation induced by both hypoxic and hypercapnic effects.
Eight men performed three series of 5-min exercise on a cycle ergometer at 65% of normoxic maximal O(2) consumption in four conditions: (1) voluntary hypoventilation (VH) in normoxia (VH(0.21)), (2) VH in hyperoxia (inducing hypercapnia) (inspired oxygen fraction [F(I)O(2)] = 0.29; VH(0.29)), (3) normal breathing (NB) in hypoxia (F(I)O(2) = 0.157; NB(0.157)), (4) NB in normoxia (NB(0.21)).
Using near-infrared spectroscopy, changes in concentration of oxy-(Delta[O(2)Hb]) and deoxyhemoglobin (Delta[HHb]) were measured in the vastus lateralis muscle. Delta[O(2)Hb – HHb] and Delta[O(2)Hb + HHb] were calculated and used as oxygenation index and change in regional blood volume, respectively. Earlobe blood samples were taken throughout the exercise.
Both VH(0.21) and NB(0.157) induced a severe and similar hypoxemia (arterial oxygen saturation [SaO(2)] < 88%) whereas SaO(2) remained above 94% and was not different between VH(0.29) and NB(0.21). Arterialized O(2) and CO(2) pressures as well as P50 were higher and pH lower in VH(0.21) than in NB(0.157), and in VH(0.29) than in NB(0.21). Delta[O(2)Hb] and Delta[O(2)Hb – HHb] were lower and Delta[HHb] higher at the end of each series in both VH(0.21) and NB(0.157) than in NB(0.21) and VH(0.29). There was no difference in Delta[O(2)Hb + HHb] between testing conditions. [La] in VH(0.21) was greater than both in NB(0.21) and VH(0.29) but not different from NB(0.157).
Woorons X, Bourdillon N, Vandewalle H, Lamberto C, Mollard P, Richalet JP, Pichon A Exercise with hypoventilation induces lower muscle oxygenation and higher blood lactate concentration: role of hypoxia and hypercapnia. European Journal of Applied Physiology. 2010 Sep;110(2):367-77.
- INTERMITTENT BREATH HOLDING PROVOKES CONSISTENT CHANGES IN MUSCLE OXYGENATION, LEADING TO LOWER TISSUE OXYGENATION
This study examined the effect of intermittent breath holding (IBH) on physiological response, including oxygenation in working muscle, to moderate-intensity exercise.
Thirteen men performed bicycle exercise for 5 min at 65% of peak oxygen uptake with normal breathing (NB) and with IBH. Muscle oxygenation, concentration changes of oxyhemoglobin (ΔOxy-Hb), deoxyhemoglobin (ΔDeoxy-Hb) and total hemoglobin (ΔTotal-Hb), in the right vastus lateralis were continuously monitored using near-infrared spectroscopy (NIRS). Finger capillary blood samples were taken after exercise for analyzing blood lactate concentration (BLa).
NIRS parameters showed acute changes to each BH episode in the IBH condition (Total-Hb and ΔOxy-Hb decreased, ΔDeoxy-Hb increased). Accordingly, in the IBH condition, ΔOxy-Hb was lower (P<0.05) and ΔDeoxy-Hb was higher (P<0.05) compared to that in the NB condition, whereas there was no difference in ΔTotal-Hb in the both conditions. BLa levels were greater (P<0.05) in the IBH condition compared to the NB condition.
These results suggest that IBH during moderate-intensity exercise provokes consistent changes in muscle oxygenation, leading to lower tissue oxygenation. Our data also indicates that exercise with IBH induces higher BLa.
Kume D, Akahoshi S, Song J, Yamagata T, Wakimoto T, Nagao M, Matsueda S, Nagao N. Intermittent breath holding during moderate bicycle exercise provokes consistent changes in muscle oxygenation and greater blood lactate response. J Sports Med Phys Fitness. 2013 Jun;53(3):327-35.
- VOLUNTARY REDUCED EXERCISE INDUCED BLOOD ACIDOSIS.
This study investigated the effects of training with voluntary hypoventilation (VH) at low pulmonary volumes. Two groups of moderately trained runners, one using hypoventilation (HYPO, n = 7) and one control group (CONT, n = 8), were constituted.
In each session, HYPO ran 24 min at 70% of maximal O2 consumption (VO2 max) with a breath holding at functional residual capacity whereas CONT breathed normally. A VO2 max and a time to exhaustion test (TE) were performed before (PRE) and after (POST) the training period.
The results of this study demonstrate that VH training did not improve endurance performance but could modify the glycolytic metabolism. The reduced exercise-induced blood acidosis in HYPO could be due to an improvement in muscle buffer capacity. This phenomenon may have a significant positive impact on anaerobic performance.
Xavier Woorons,, Pascal Mollard a, Aur´elien Pichon a, Alain Duvallet, Jean-Paul Richalet, Christine Lamberto. Effects of a 4-week training with voluntary hypoventilation carried out at low pulmonary volumes. Respiratory Physiology & Neurobiology 160 (2008) 123–130
- HYPOVENTILATION AT LOW LUNG VOLUME IMPROVED REPEATED SPRINT ABILITY IN SWIMMING
Repeated-sprint training in hypoxia (RSH) has been shown as an efficient method for improving repeated sprint ability (RSA) in team-sport players but has not been investigated in swimming. We assessed whether RSH with arterial desaturation induced by voluntary hypoventilation at low lung volume (VHL) could improve RSA to a greater extent than the same training performed under normal breathing (NB) conditions.
16 competitive swimmers completed six sessions of repeated sprints (two sets of 16×15 m with 30 s send-off) either with VHL (RSH-VHL, n=8) or with NB (RSN, n=8). Before (pre-) and after (post-) training, performance was evaluated through an RSA test (25m all-out sprints with 35 s send-off) until exhaustion.
From pre- to post-, the number of sprints was significantly increased in RSH-VHL (7.1 ± 2.1 vs 9.6 ± 2.5; p<0.01) but not in RSN (8.0 ± 3.1 vs 8.7 ± 3.7; p=0.38). Maximal blood lactate concentration ([La]max) was higher at post- compared to pre- in RSH-VHL (11.5 ± 3.9 vs 7.9 ± 3.7 mmol.l-1; p=0.04) but was unchanged in RSN (10.2 ± 2.0 vs 9.0 ± 3.5 mmol.l-1; p=0.34). There was a strong correlation between the increases in the number of sprints and in [La]max in RSH-VHL only (R=0.93; p<0.01). Repeated sprint training in hypoxia induced by voluntary hypoventilation at low lung volume improved repeated sprint ability in swimming, probably through enhanced anaerobic glycolysis. This innovative method allows inducing benefits normally associated with hypoxia during swim training in normoxia.
Trincat L, Woorons X, Millet GP. Repeated Sprint Training in Hypoxia Induced by Voluntary Hypoventilation in Swimming. Int J Sports Physiol Perform. 2016 Jun 13.
- VOLUNTARY HYPOVENTILATION DURING EXERCISE AUGMENTS ELECTROMYOGRAPHY (EMG) ACTIVITY
It has been reported that exercise under hypoxic conditions induces reduced muscle oxygenation, which could be related to enhanced activity on electromyography (EMG).
Although it has been demonstrated that exercise under conditions of voluntary hypoventilation (VH) evokes muscle deoxygenation, it is unclear whether VH during exercise impacts EMG. Seven men performed bicycle exercise for 5 min at 65 % of peak oxygen uptake with normal breathing (NB) and VH.
Muscle oxygenation; concentration changes in oxyhemoglobin (Oxy-Hb), deoxyhemoglobin (Deoxy-Hb) and total hemoglobin (Total-Hb); and surface EMG in the vastus lateralis muscle were simultaneously measured.
In the VH condition, Oxy-Hb was significantly lower and Deoxy-Hb was significantly higher compared to those in the NB condition (P < 0.05 for both), whereas there was no significant difference in Total-Hb between the two conditions.
We observed significantly higher values (P < 0.05) on integrated EMG during exercise under VH conditions compared to those under NB conditions. This study suggests that VH during exercise augments EMG activity.
Kume D, Akahoshi S, Yamagata T, Wakimoto T, Nagao N. Does voluntary hypoventilation during exercise impact EMG activity? Springerplus. 2016 Feb 24;5:149.