Science / Aerobic Capacity: How to Increase, Exercises, Benefits
Aerobic Capacity: How to Increase, Exercises, Benefits
How to Access the Spleen’s Rich Blood Bank to Boost Your Body’s Oxygen Capacity
In this chapter we’ll learn:
- What is aerobic capacity?
- What is “hematocrit” and why is it important?
- How to increase your aerobic capacity
- Why better aerobic capacity benefits athletic performance (and health)
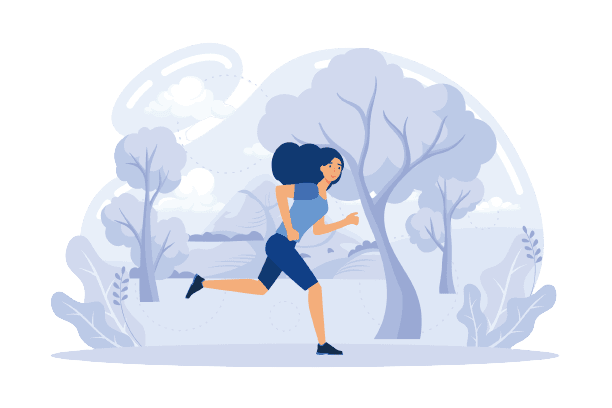
During aerobic exercise, your heart pumps oxygen-loaded blood to your working muscles. The oxygen helps you burn fat and carbohydrate for energy. In fact, “aerobic” literally means “with oxygen” where “anaerobic” means “without oxygen.”
We’ve already looked at anaerobic capacity in our Science series. But what about aerobic threshold? Aerobic capacity is a measure of cardiorespiratory fitness. It reflects your general state of health. It’s your maximal oxygen uptake during physical activity – your VO2 max.
A high VO2 max represents greater aerobic power and endurance. With better aerobic endurance you can run, cycle or swim at a moderate intensity for longer. In other words, with a higher VO2 max, your body’s oxygen consumption is more efficient. You can burn more fuel and work out for longer.
Oxygen travels round the body in hemoglobin. Hemoglobin is a protein molecule in red blood cells. The percentage of red blood cells in your blood is called your hematocrit level. The normal range for hematocrit is different for men and women. In healthy females it’s between 36 and 44%, and it’s 41 to 50% in males.
Hematocrit levels increase when the spleen contracts. The spleen is the body’s blood bank. Its function is to hold onto excess blood. If demand is high, it pumps more red blood cells into circulation. The blood in the spleen is densely packed with around 80% oxygen-carrying red blood cells.
During a strong breath hold, the body senses a drop in blood oxygen saturation. The spleen responds by contracting to release more red blood cells into circulation. This boosts your hematocrit. Which increases the oxygen-carrying capacity of your blood by between 2.8 and 9.6%. If your blood is able to carry more oxygen, your aerobic capacity is greater.
This is important. Aerobic capacity is a major differentiator in endurance events. Poor aerobic capacity can make it difficult to keep up with the competition. And in health terms, it’s a bigger risk for early death than high blood pressure or high cholesterol.
How Can I Increase my Aerobic Capacity?
It’s a common misconception that if you want more oxygen, you should breathe more air. The opposite is true. In fact, breath holds or “hypoventilation” training (reduced breathing) improve body oxygenation.
First, you must make the switch to full-time nose breathing. This will improve your tolerance to carbon dioxide. Better CO2 tolerance means your body can use oxygen more efficiently.
Nasal breathing adds resistance to airflow during inhalation and exhalation. This allows better uptake of oxygen into the blood. And better oxygenation of the muscles, tissues and organs.
Nose breathing also harnesses nitric oxide (NO). NO dilates the airways and blood vessels in the lungs. Again, it means better oxygen uptake.
Breath holds to simulate training at high altitude (hypoxic/hypercapnic training) are also beneficial. When you practice strong breath holds, you can access the hematocrit-rich blood in your spleen.
Just 5 breath holds of 30 seconds each will trigger splenic contractions. This causes the spleen to release more red blood cells. Which boosts your aerobic capacity.
Strong breath holds to boost hematocrit are safe, legal and ethical. Giving you a natural advantage.
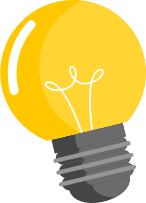
Benefits of Increased Aerobic Capacity for Performance
- More stamina
- Increased energy
- Healthy blood pressure
- Lower cholesterol
- Better blood sugar regulation
- You burn more calories
- Body weight regulation
- Greater mental alertness
- A calm mind before competition
- And, muscle changes, including greater muscle mass and mitochondrial density. The mitochondria are the parts of the cells that convert oxygen into fuel. More mitochondria means your muscles can use oxygen better. Better mitochondrial density also improves the health and function of your cells.
Hypoxic/hypercapnic training replicates aspects of training at high altitude. But it also has other advantages. The physiological adaptations caused by “real” elevation training only last two weeks. But simulation of high altitude training is readily accessible. After all, you can practice a breath hold any time you need!
N.B. When practicing strong breath holds to achieve hypoxia/hypercapnia, take a 2-minute break between each breath hold. This allows CO2 to normalize. If CO2 is too high, you will struggle to maintain a long breath hold.
What the Scientists Say about Aerobic Capacity
- 10.79% INCREASE TO VO2 MAX, 5.35% INCREASE TO HEMOGLOBIN
Research was conducted to establish the effects of 8 week hypercapnic-hypoxic training program in elite male swimmers, 30 to 45 minutes, three times a week. Each test subject has withheld breath individually, by a subjective feeling, for as long as possible. The condition is that each breath hold must be above the minimum values which describe hypercapnia, that is, the values of carbon dioxide in the exhaled breath had to be over 45 mmHg, which was controlled by a capnometer.
Besides the swimming training sessions the control group was subjected to additional aerobic training sessions on a treadmill. The program was conducted three times a week for eight weeks.
Experiment Control
Pre: Hb (g/L) 144.63 147.75
Post: Hb (g/L) 152.38 145.38
The above shows a 5.35% increase to hemoglobin in the group that practiced breath holding after an exhalation. Furthermore, there was a 10.79% increase to VO2 max as shown below:
Experiment Control
VO2 Max Pre: 63.80 59.46
VO2 Max Post: 70.38 60.81
See: Dajana Zoretic, Nada Grcic-Zubcevic and Katarina Zubcic. “THE EFFECTS OF HYPERCAPNIC-HYPOXIC TRAINING PROGRAM ON HEMOGLOBIN CONCENTRATION AND MAXIMUM OXYGEN UPTAKE OF ELITE SWIMMERS”. Faculty of Kinesiology, University of Zagreb, Croatia.
- HEMATOCRIT (HCT) INCREASE BY 6.4% AND HEMOGLOBIN CONCENTRATION (HB) INCREASE BY 3.3%
Results found that the volunteers with spleens showed a 6.4 percent increase in hematocrit (Hct) and a 3.3 percent increase in hemoglobin concentration (Hb) following the breath holds. This means that after just five breath holds, the oxygen-carrying capacity of the blood was significantly improved.
However, for the individuals who had their spleens removed (due to prior medical reasons), there were no recorded changes to the blood resulting from breath holding.
Schagatay E, Andersson JP, Hallén M, Pålsson B.. Selected contribution: role of spleen emptying in prolonging apneas in humans. Journal of Applied Physiology.2001;(Apr;90(4)):1623-9
- HEMATOCRIT (HCT) INCREASE BY 4%
Seven male volunteers performed two sets of five breath holds to near maximal duration; one in air and the other with their faces immersed in water. Each breath hold was separated by two minutes of rest and each set separated by twenty minutes.
Both Hct and Hb concentration increased by approximately 4 percent across both series of breath holds – in air and in water. This study in particular provides pertinent information about the consequence of breath holding: since there was no visible increase in the results of breath holding with the subject’s faces immersed in water, the authors concluded that “the breath hold, or its consequences, is the major stimulus evoking splenic contraction.”
See: Schagatay E, Andersson JP, Nielsen B. Hematological response and diving response during apnea and apnea with face immersion. European Journal of Applied Physiology.2007;(Sep;101(1):):125-32
- SPLEEN SIZE DECREASED BY A TOTAL OF 20 PERCENT
A study by Baković et al from University of Split School of Medicine, Croatia, was conducted to investigate spleen responses resulting from five maximal breath holds. Ten trained breath hold divers, ten untrained volunteers and seven volunteers who had their spleen removed were recruited. The subjects performed five maximum breath holds with their face immersed in cold water, and each breath hold was separated by a two-minute rest.
The duration of the breath holds peaked at the third attempt, with breath hold divers reaching 143 seconds, untrained divers reaching 127 seconds and splenectomised persons achieving 74 seconds. Spleen size decreased by a total of 20 percent in both breath hold divers, and the untrained volunteers.
Researchers concluded that the “results show rapid, probably active contraction of the spleen in response to breath hold in humans. Rapid spleen contraction and its slow recovery may contribute to prolongation of successive, briefly repeated breath hold attempts.”
See: Darija Baković, Zoran Valic, Davor Eterović, Ivica Vuković, Ante Obad, Ivana Marinović-Terzić, Zeljko Dujić. Spleen volume and blood flow response to repeated breath-hold apneas. Journal of Applied Physiology.2003;(vol. 95 no. 4):1460-1466
- LONGER BREATH HOLDS CAUSES GREATER SPLENIC CONTRACTION
In a paper by Dr Espersen and colleagues from Herlev Hospital, University of Copenhagen, Denmark, splenic contraction was found to take place even with very short breath holds of 30 seconds.
However, the strongest contraction of the spleen was as it released blood cells into circulation, occurring when a subject held their breath for as long as possible.
See: Kurt Espersen, Hans Frandsen, Torben Lorentzen, Inge-Lis Kanstrup,Niels J. Christensen. The human spleen as an erythrocyte reservoir in diving-related interventions . Journal of Applied Physiology.2002;(May;92(5)):2071-9
- LONG TERM EFFECTS OF BREATH HOLDING
French researcher Lematires wrote a very interesting paper entitled ‘Apnea – A new training method in sport’ in which he noted that resting Hb mass in trained breath hold divers was 5 percent higher than in untrained divers.
In addition, breath hold divers showed a larger relative increase to Hb after three apneas. The paper noted that, “the long-term effect of apnea training on Hb mass might be implicated in elite divers’ performance.”
See: Lemaître F, Joulia F, Chollet D. Apnea: a new training method in sport? Med Hypotheses.2010;(Mar;74(3)):413-5
- INCREASE OF HEMOGLOBIN CONCENTRATION AFTER MAXIMAL APNEAS IN DIVERS, SKIERS, AND UNTRAINED HUMANS
Matt Richardson investigated the haematological responses to maximal apneas performed by three groups: elite apnoeic divers, elite cross country skiers and untrained subjects. Pre-test hemoglobin tended to be higher in the diver group than both skiers and untrained individuals.
Each subject was required to perform three maximal breath holds separated by two minutes of rest and normal breathing. Following the breath holds, all groups responded with increased hemoglobin, with divers showing the largest increase. The duration of the third breath hold time was 187 seconds in divers, 111 seconds in skiers, and 121 seconds in untrained individuals.
The authors observed that the higher Hb concentration in divers “suggests that regular apnea practice could impart a specific training effect, effecting haematological responses to apnea in a manner that differs from that of exercise training.”
See: Richardson M, de Bruijn R, Holmberg HC, Björklund G, Haughey H, Schagatay E. Increase of hemoglobin concentration after maximal apneas in divers, skiers, and untrained humans. Canadian Journal Applied Physiology.2005;(Jun;30(3)):276-81
- HEMATOCRIT INCREASED 9.5 PERCENT
Splenic size was measured before and after repetitive breath hold dives to approximately 6 meters in ten Korean ama (diving women) and in three Japanese males who were not experienced in breath holding. Following the breath holds, splenic size and hematocrit were unchanged in the Japanese male divers.
In the ama, splenic volume decreased 19.5 percent, hemoglobin increased by 9.5 percent, and hematocrit increased 9.5 percent. The study showed that long-term repeated apneas induce a stronger spleen contraction and resultant hematological response.
See: Hurford WE, Hong SK, Park YS, Ahn DW, Shiraki K, Mohri M, Zapol WM. Splenic contraction during breath-hold diving in the Korean ama. Journal Applied Physiology.1990;(Sep;69(3)):932-6
- NONHEMATOLOGICAL MECHANISMS OF IMPROVED SEA-LEVEL PERFORMANCE AFTER HYPOXIC EXPOSURE
Specific beneficial nonhematological factors include improved muscle efficiency probably at a mitochondrial level, greater muscle buffering, and the ability to tolerate lactic acid production.
This review explores evidence of factors other than accelerated erythropoiesis that can contribute to improved athletic performance at sea level after living and/or training in natural or artificial hypoxia. We describe a range of studies that have demonstrated performance improvements after various forms of altitude exposures despite no increase in red cell mass.
In addition, the multifactor cascade of responses induced by hypoxia includes angiogenesis, glucose transport, glycolysis, and pH regulation, each of which may partially explain improved endurance performance independent of a larger number of red blood cells.
Specific beneficial nonhematological factors include improved muscle efficiency probably at a mitochondrial level, greater muscle buffering, and the ability to tolerate lactic acid production. Future research should examine both hematological and nonhematological mechanisms of adaptation to hypoxia that might enhance the performance of elite athletes at sea level.
See: Gore CJ, Clark SA, Saunders PU. Nonhematological mechanisms of improved sea-level performance after hypoxic exposure. Med Sci Sports Exerc. 2007 Sep;39(9):1600-9.
- NOT ALL RESEARCHERS HAVE REPORTED IMPROVEMENTS TO AEROBIC CAPACITY. MORE RESEARCH IS REQUIRED.
This study investigated the effects of training with voluntary hypoventilation (VH) at low pulmonary volumes. Two groups of moderately trained runners, one using hypoventilation (HYPO, n=7) and one control group (CONT, n=8), were constituted.
The training consisted in performing 12 sessions of 55 min within 4 weeks. In each session, HYPO ran 24 min at 70% of maximal O(2) consumption ( [V(02max)) with a breath holding at functional residual capacity whereas CONT breathed normally. A V(02max) and a time to exhaustion test (TE) were performed before (PRE) and after (POST) the training period.
The results of this study demonstrate that VH training did not improve endurance performance but could modify the glycolytic metabolism. The reduced exercise-induced blood acidosis in HYPO could be due to an improvement in muscle buffer capacity. This phenomenon may have a significant positive impact on anaerobic performance.
See: Xavier Woorons , Pascal Mollard, Aur´elien Pichon, Alain Duvallet, Jean-Paul Richalet, Christine Lamberto. Effects of a 4-week training with voluntary hypoventilation carried out at low pulmonary volumes. Respiratory Physiology & Neurobiology 160 (2008) 123–130.